Data-driven materials design: Artificial Intelligence as a tool supporting the synthesis of nanocomposites with hierarchical porosity
The demand for advanced materials for “clean energy production” is constantly growing and is associated with the search for safer, cheaper, and more ecological solutions. Among these sought advanced materials are compounds for basic processes of future technologies, including those with catalytic properties. Catalytic properties are understood here as those accelerating chemical reactions, among other things, by lowering its energy barrier, i.e., the barrier that must be overcome to initiate the course of the reaction. In particular, researchers and industry practitioners are interested in materials that can be used for electrocatalytic reactions, i.e., those in electrochemical systems at the electrode/solution interface. One such electrocatalytic reaction is the oxygen reduction reaction (ORR), an energy source in devices such as fuel cells, microbial fuel cells and metal-air batteries. Currently, the research on electrocatalytic processes is dominated by materials based on noble metals, such as platinum and palladium. However, they are characterized by low durability, susceptibility to gas poisoning, low activity and selectivity. The second group of materials popular in electrocatalysis is carbon materials with a large number of pores, in which the main limitations in achieving high operational efficiency are, among others, the heterogeneous structure of their surface, high content of impurities, low resistance to oxidation and inefficient transport of electrons and heat. Motivated by these challenges, as part of the project, we plan to synthesize nanocomposite materials containing cyclic skeletons with nitrogen atoms in their structure and spherical carbon nanostructures (multi-layered fullerenes), which should be characterized by high electrocatalytic activity. This idea is unique because nanocomposites of this type have yet to be used in electrocatalytic reactions. Our research shows that nanocomposite materials have properties such as high electronic conductivity, a large number and increased accessibility of active sites for reactants, high porosity, which enables easy transport of electrons, and high chemical and electrochemical durability. As part of the project, we plan to design highly efficient nanocomposite catalysts with particular emphasis on the correlation between the porous structure of materials and the efficiency of the electrocatalytic reaction and to design advanced materials for electrocatalysis using the “lab-in-the-loop” approach based on artificial intelligence (AI). Our main goal is to revolutionize the synthesis methodology by developing an AI-based approach to catalyst design to improve the electrocatalytic performance of materials and achieve outstanding performance parameters.
As part of this project, we intend to combine experimental work in obtaining materials for electrocatalysis with AI in the form of machine learning (ML) algorithms. Using ML to find the relationship between the structural parameters of the substrate, product and electrocatalytic performance may favor the design of new systems with advanced properties. We call our approach data-driven material design. This combination of ML and synthesis is entirely original and, to our knowledge, has never been used in materials chemistry in the context of substrates for electrocatalytic reactions. Using an innovative “lab-in-the-loop” approach, we can effectively design advanced materials for electrocatalysis.
Project: OPUS 2023/49/B/ST5/02403 (2024-present), NCN, Poland; entitled “Data-driven materials design: Artificial Intelligence as a tool supporting the synthesis of nanocomposites with hierarchical porosity for electrocatalytic applications.”
Porous materials
The unique physicochemical properties of porous materials determine their undying interest in many fields of science. Their specific properties are mainly related to their structure – porous structure (among others, number, shape, size, distribution in the material, and interconnection of pores). Multidisciplinary research focuses on synthesizing resins, oligomers and polymers and their use as a matrix in porous nanocomposites. We are using porous materials where it is necessary to search for the materials with an expanded active surface, extremely high porosity, mainly related to the presence of the micro- and macropores, low thermal conductivity and high dielectric permittivity. Due to their multi-functionality, they have a variety of potential applications, including fuel cells, capacitors and dielectrics, elastomers, electrochemical sensors and sensors for immobilizing enzymes or biologically active compounds (biosensors).
Project: OPUS 2017/25/B/ST5/01414 (2018-2022), NCN, Poland; entitled “Synthesis of porous nanocomposite materials, their physicochemical properties and application in electrochemistry.”
Sci Rep 2023, 13, 6606, Composites containing resins and carbon nano-onions as efficient porous carbon materials for supercapacitors, https://doi.org/10.1038/s41598-023-33874-w.
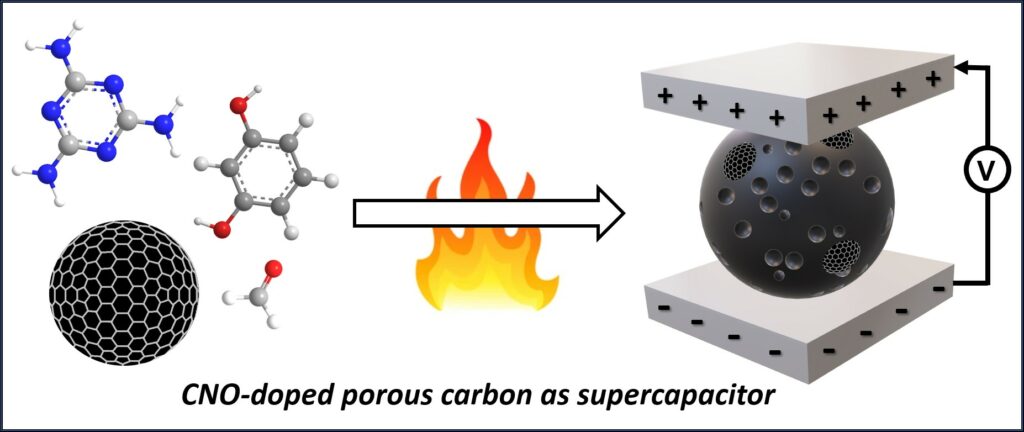
Chem. Comm. 2022, 58, 6829-6832, Carbon nano-onion induced organization of polyacrylonitrile-derived block star polymers to obtain mesoporous carbon materials, https://doi.org/10.1039/D2CC01452A
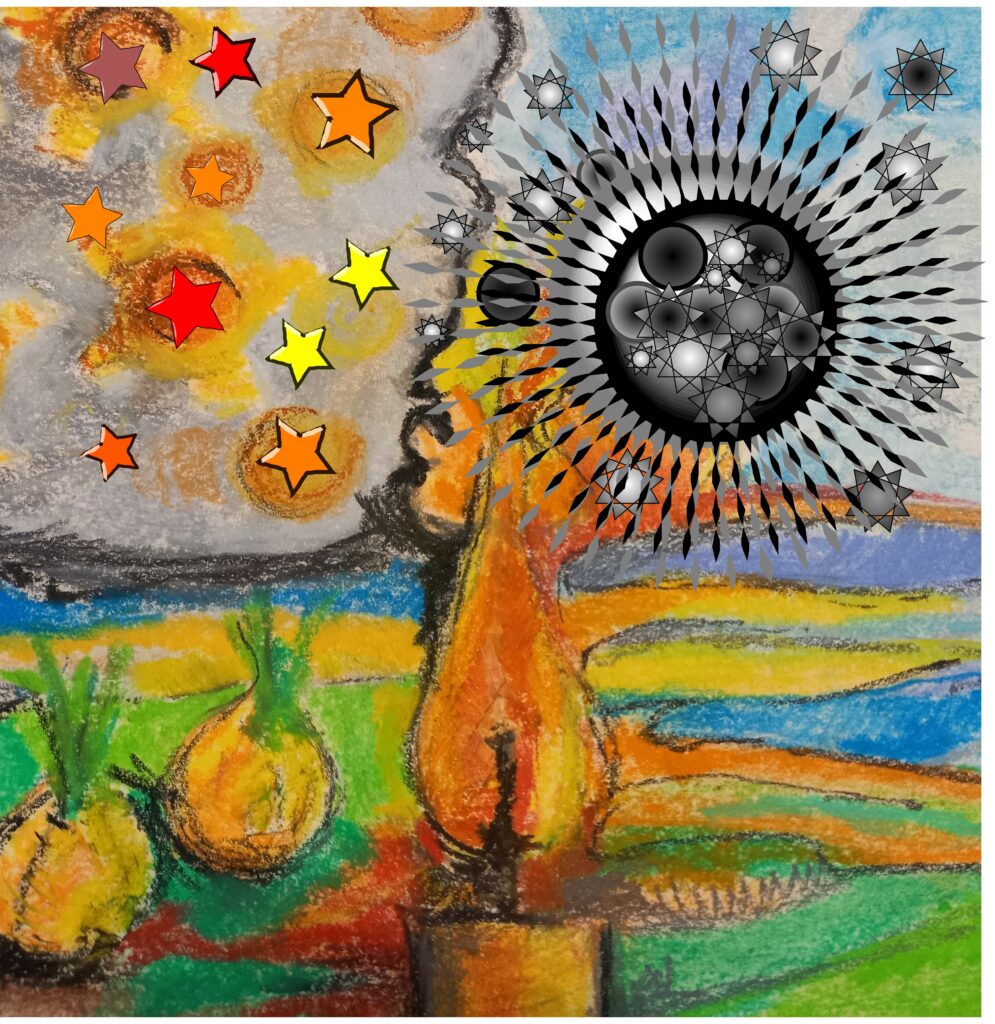
ACS Appl. Polym. Mater. 2022, 4, 4, 2442–2458, Polymeric Network Hierarchically Organized on Carbon Nano-onions: Block Polymerization as a Tool for the Controlled Formation of Specific Pore Diameters, https://doi.org/10.1021/acsapm.1c0
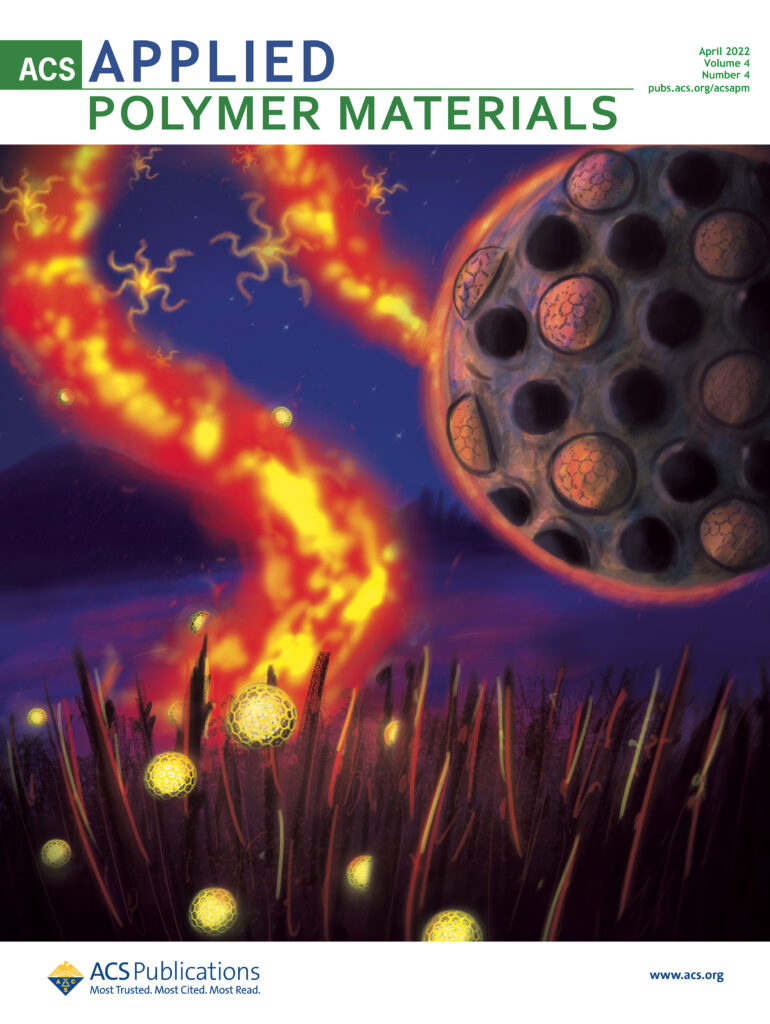
J. Mater. Chem. A 2018, 6, 845-852, A phenol-formaldehyde polymeric network to generate organic aerogels: synthesis, physicochemical characteristics and potential applications, https://doi.org/10.1039/C7TA08814K
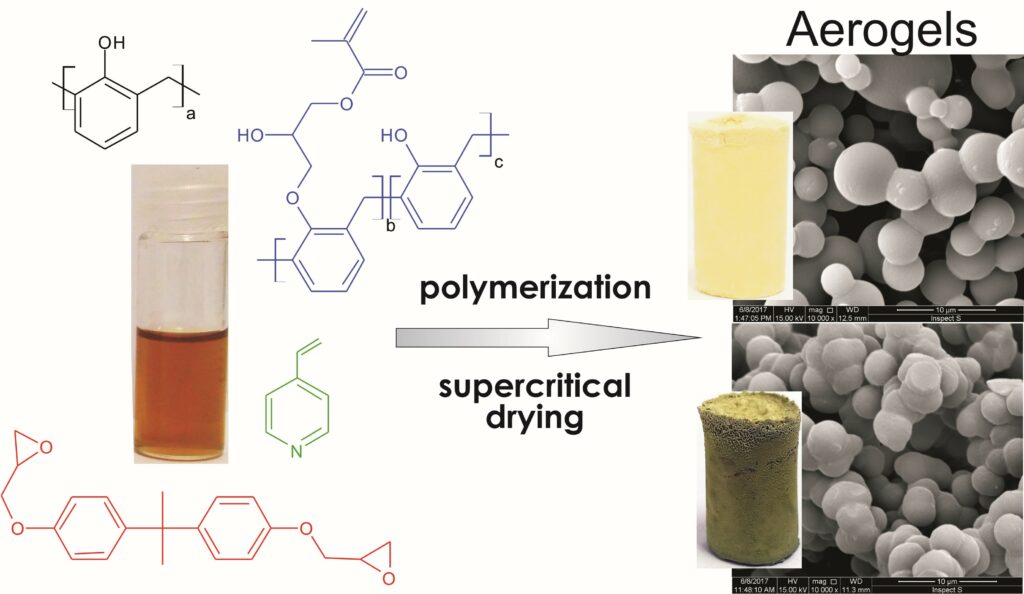
Defective carbon nanostructures
Developing advanced catalysts for efficient electrochemical energy conversion has attracted much interest in recent decades. Catalysts reduce the energy needed to start the reaction and continue the process; therefore, their use in industry is essential for many devices’ efficient and inexpensive operation. Since then, the global study of electrocatalytic processes has been dominated by materials based on noble metals, such as platinum, palladium, molybdenum, etc. Generally, metal catalysts are characterized by low selectivity, poor durability and susceptibility to gas poisoning. In addition, due to the cost and rarity of some metals, their practical use on a large scale still needs to be improved. In this context, searching for new solutions and alternative materials abounding on the earth is essential. Recently, a new generation of electrocatalysts has been developed, promoting materials with a highly developed surface and high defectivity. Carbon materials are ideal candidates for this purpose. Carbon materials can act as electrocatalysts due to their enlarged active surface, strongly related to their defectivity, which various experimental methods can quickly form. The nature and number of defects introduced into carbon materials are of crucial importance for the electrocatalytic activity of the materials.
Projects: 1. OPUS 2019/35/B/ST5/00572 (2020-present), NCN, Poland; entitled “Carbon electrocatalysts with structural defects: preparation, characterization and study of structure-catalytic activity relationships.” 2. SONATA BIS 2012/05/E/ST5/03800 (2013-2019), NCN, Poland; entitled “Carbon nano-onions as a new matrix for materials accumulating electric charge and photovoltaic systems – synthesis and characterization of physicochemical properties.”
ACS Appl. Nano Mater. 2022, 5, 11, 16376–16387, Nanostructured Carbon Catalyst for Amide Coupling Reactions under Microwave Heating in the Absence of a Solvent, https://doi.org/10.1021/acsanm.2c03437
The nanostructured carbon material catalyzes the direct amide coupling reaction under microwaved-assisted heating in the absence of a solvent. The absorption of microwaved radiation by the nanostructured carbon catalyst effectively influences on yield of the catalytic reaction, which is up to 94%. Additionally, the recovery of catalytic material is straightforward.
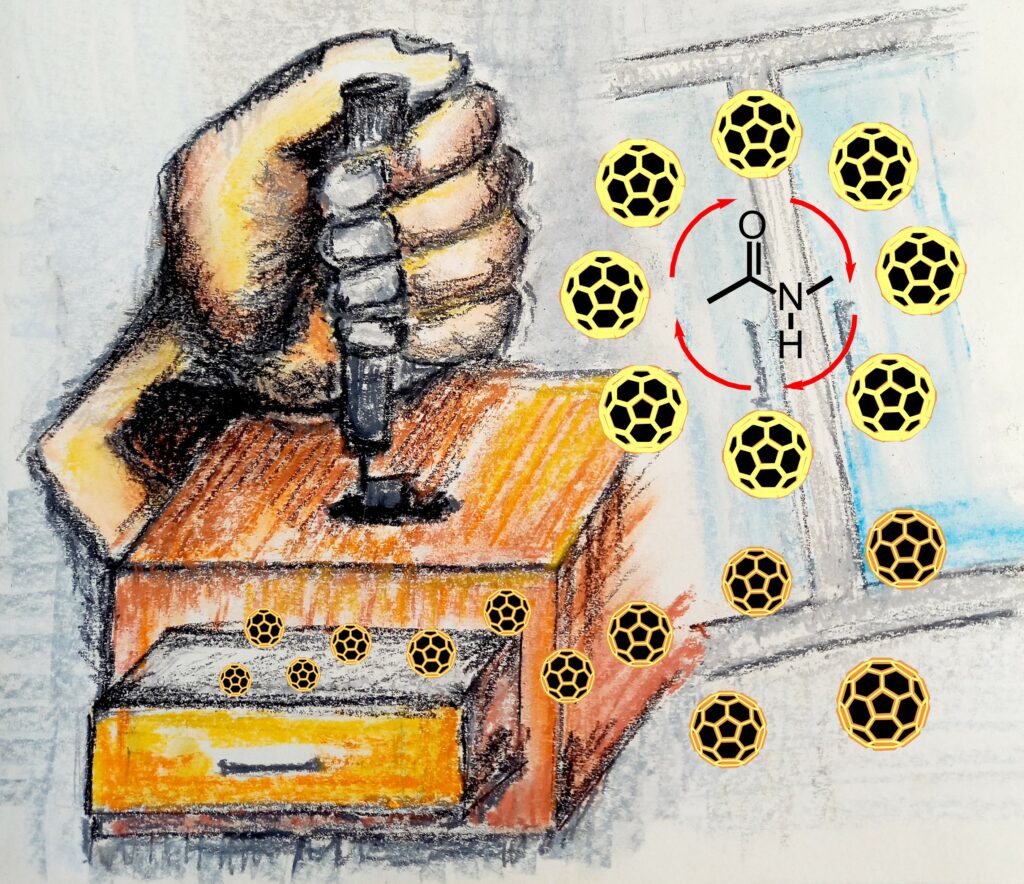
ACS Appl. Mater. Interfaces 2021, 13, 43, 51628–51642, Linking the Defective Structure of Boron-Doped Carbon Nano-Onions with Their Catalytic Properties: Experimental and Theoretical Studies, https://doi.org/10.1021/acsami.1c12126
Carbon 2019, 151, 120-129, Correlation between the catalytic and electrocatalytic properties of nitrogen-doped carbon nanoonions and the polarity of the carbon surface: Experimental and theoretical investigations, https://doi.org/10.1016/j.carbon.2019.05.069
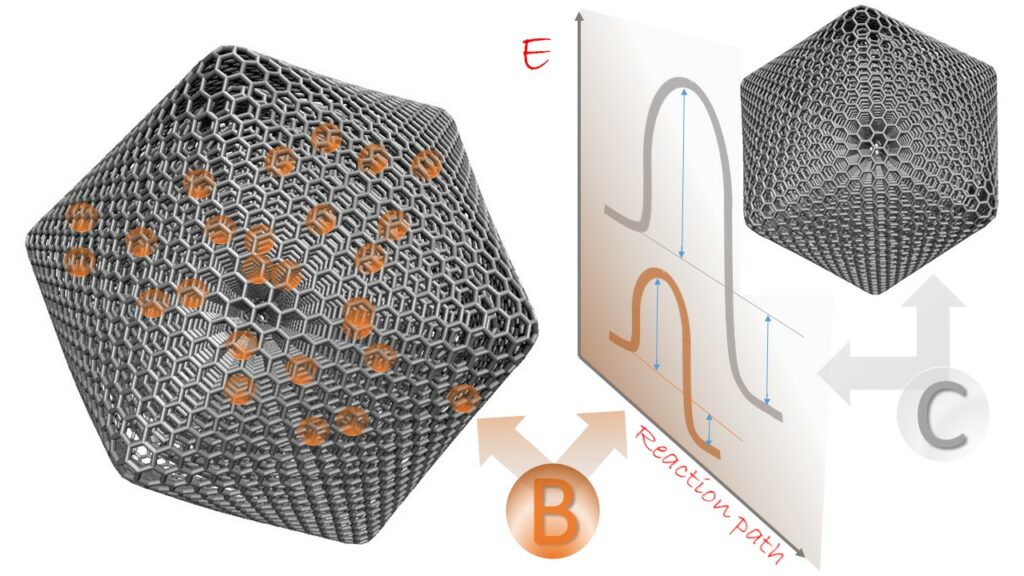
ChemNanoMat 2017, 3(8), 583-590, Improvement of the Structural and Chemical Properties of Carbon Nano-onions for Electrocatalysis, https://doi.org/10.1002/cnma.201700161
Covalent triazine-based frameworks
Covalent triazine-based frameworks (CTFs) have attracted much interest in recent years due to their high surface area and excellent thermal and electrochemical stabilities. Their synthesis relies on forming a triazine ring as a covalent bond between organic building blocks to achieve an extended porous framework. Due to the stable triazine-based linkage, high intrinsic nitrogen content, and ability to add heteroatoms to their structures, CTFs have the potential to be utilized in several applications, such as photo- and electrocatalysis, batteries, gas adsorption, separation, and pollutant removal. The main advantage of CTFs is that a regular two-dimensional organization is obtained without using auxiliary structure-directing agents. The pore size can be adjusted by simply tuning the size and structural features of the starting monomer.
Scientific Reports 2023, 13, 10737. Three-dimensional organization of pyrrolo[3,2-b]pyrrole-based triazine framework using nanostructural spherical carbon: enhancing electrochemical performance of materials for supercapacitors. DOI: https://doi.org/10.1038/s41598-023-37708-7
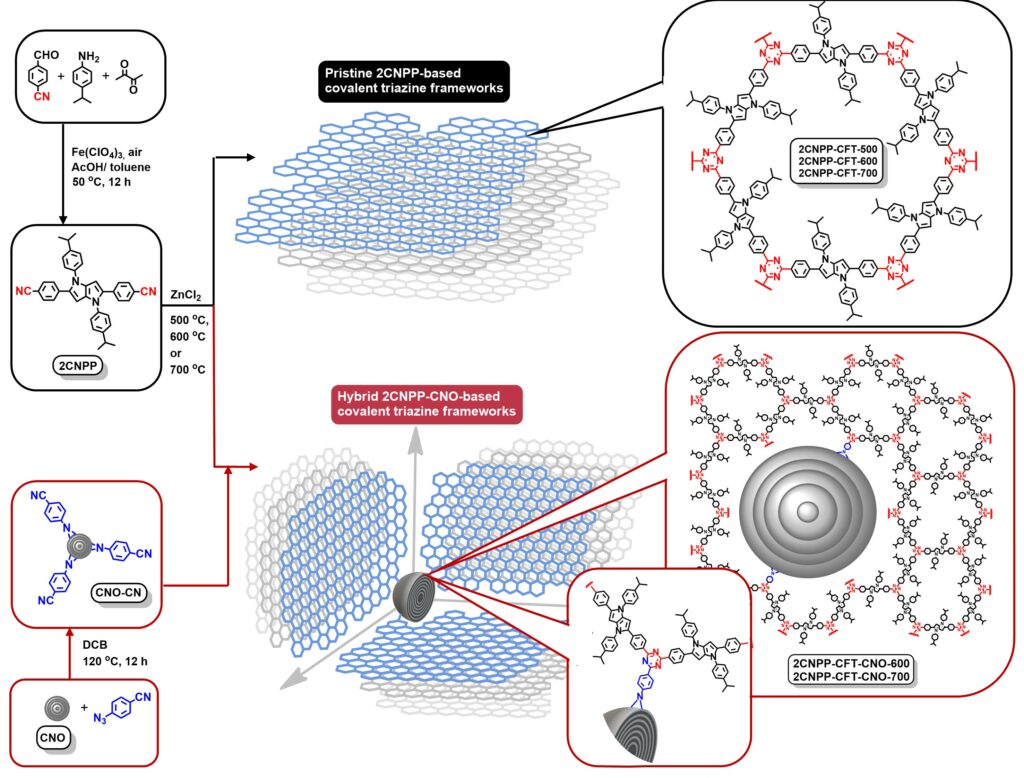
ChemRxiv, Hierarchical porosity of hybrid carbon nanomaterials based on a covalent triazine framework for high-performance capacitive energy storage, DOI: 10.26434/chemrxiv-2022-qpgjj
Carbon nano-onions: properties and applications
Among the many allotropic forms of carbon, we can distinguish multi-layered fullerenes, often called “carbon nano-onions” (CNOs). The name is closely related to their spherical structure and their multi-layered structures. These structures’ physical and chemical properties are strongly connected with their size and the number of graphene layers in the nanostructures. The unique combination of high electrical conductivity (comparable to carbon black), large external surface area, and nanoscopic size, alongside the possibility for large-scale synthesis and chemical modification, has made this material very attractive for our applications, ranging from electrochemical energy storage, electrocatalysis, to biomedical applications.
The CNO structure consists mainly of hexagonal rings like graphite layers but also contains pentagonal and heptagonal rings and occasional quadrangular and octagonal rings recognized as surface defects. These bonds range from 1.455 to 1.460 Å for a CC bond between a hexagon and a pentagon and from 1.400 to 1.391 Å for a CC bond between two hexagons, similar to monolayer fullerenes. Although the chemical bonds between carbon atoms are similar (the bonds are considered double bonds), different carbon nanostructures do not exhibit the same reactivity. It is known that the reactivity of fullerenes is partly dictated by the degree of surface deformation: the greater the curvature, the greater the reactivity. This property is critical in fullerene chemistry. Analogously to other carbon nanostructures, CNOs in their original (unmodified) form show very low dispersibility in polar and non-polar solvents. However, their small size allows for easy surface functionalization compared to other large and “flatter” carbon nanostructures. Therefore, various approaches use covalent or supramolecular strategies such as oxidation, 1,3-dipolar cycloaddition, amidation, cycloaddition, nucleophilic substitution, diazonium addition, reduction, and reduction alkylation, and radical polymerization have been used to dissolve CNO. Despite their easy functionalization, one of the most exciting properties of CNOs is their conductivity. CNOs formed at high temperatures (~1800 °C) show the best properties in terms of conductivity. The combination of high intrinsic electrical conductivity and easy ion accessibility through the outer surface makes CNOs attractive for high-power applications requiring a high charge and discharge rates. Using broadband dielectric spectroscopy, we analyzed the electrical properties of conductive composites containing CNOs. We found that the enormous effective surfaces of the CNOs acted as multi-layer concentric spherical capacitors and probably induced a large capacitance.
Projects: 1. SONATA BIS 2012/05/E/ST5/03800 (2013-2019), NCN, Poland; entitled “Carbon nano-onions as a new matrix for materials accumulating electric charge and photovoltaic systems – synthesis and characterization of physicochemical properties.” 2. OPUS 2011/01/B/ST5/06051 (2011-2014), NCN, Poland; entitled “Functionalization of “small” carbon nano-onions with polyphenolic compounds and their potential application in elastin/collagen biosensors.”
RSC Advances 2020,10, 10910-10920, Nanostructural catalyst: metallophthalocyanine and carbon nano-onion with enhanced visible-light photocatalytic activity towards organic pollutants, https://doi.org/10.1039/D0RA00896F
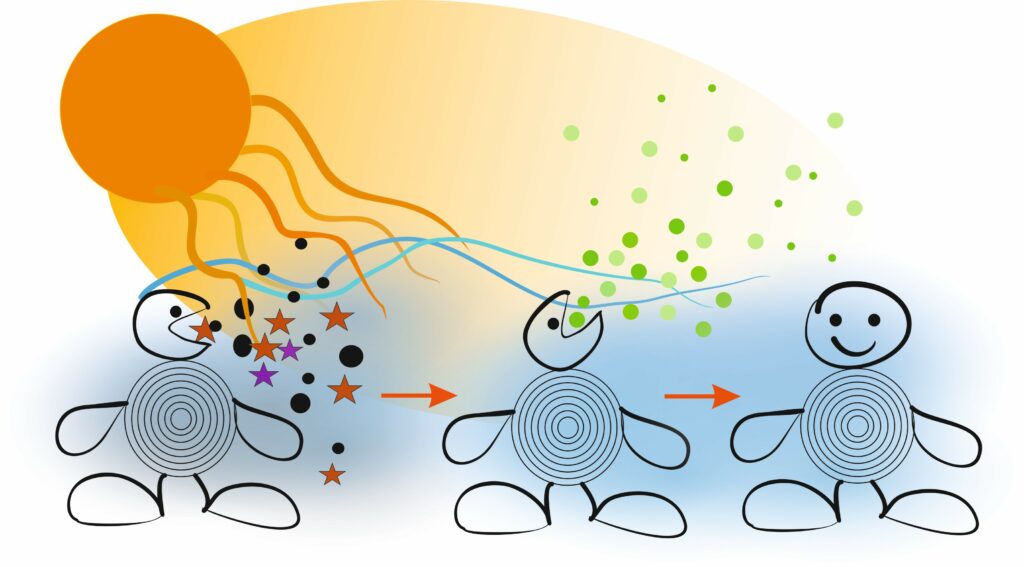
ChemNanoMat 2020, 6(2) 248-257, Carbon Nano-Onion and Zinc Oxide Composites as an Electron Transport Layer in Inverted Organic Solar Cells, https://doi.org/10.1002/cnma.201900561
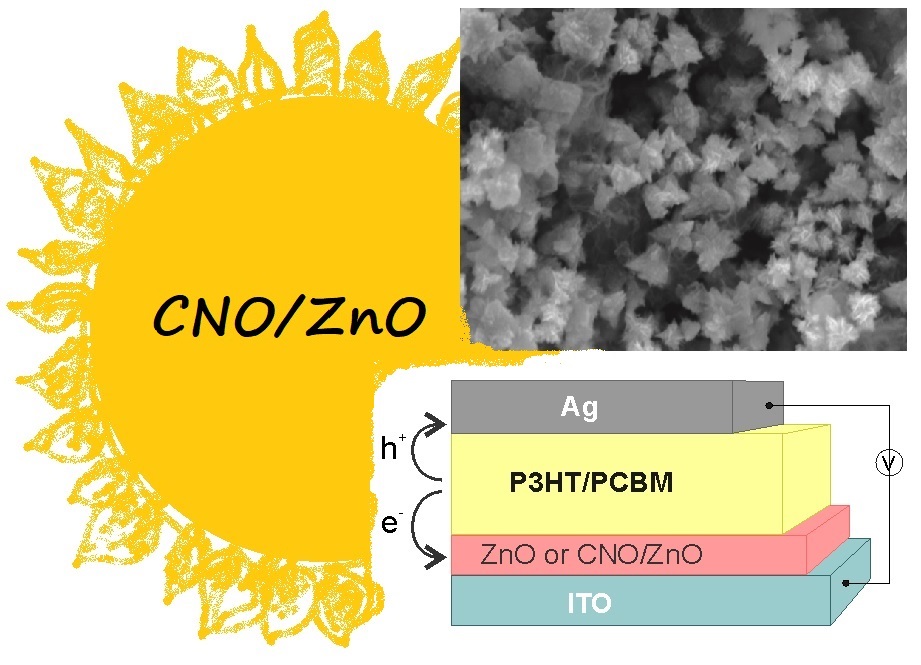
ChemNanoMat 2019, 5, 568-580, Carbon Nano-Onions: A Review of Recent Progress in Synthesis and Applications https://doi.org/10.1002/cnma.201800583
Nanoscale Advances 2019, 1, 3164-3176, Carbon nanoonion-ferrocene conjugates as acceptors in organic photovoltaic devices, https://doi.org/10.1039/C9NA00135B
Chem. Eur. J. 2017, 23(29), 7132-7141, Boron-Doped Polygonal Carbon Nano-Onions: Synthesis and Applications in Electrochemical Energy Storage, https://doi.org/10.1002/chem.201700914